Npj Comput. Mater.:强韧双全—沉淀相转化增强金属玻璃的延展性?
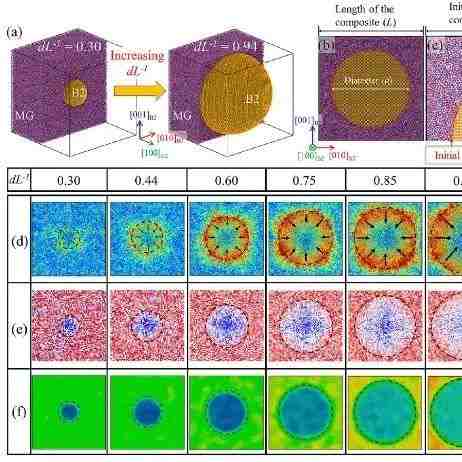
海归学者发起的公益学术平台
分享信息,整合资源
交流学术,偶尔风月
Fig. 1 The distribution of atomic displacements, volumetric stress and volumetric strain within the 3D composite model.
这些现象通常与非晶基体对可转变B2相在低温下施加的“限域效应”或“流体静压”有关,这一点是根据经典的Eshelby理论解释的。同时,界面处的应力/应变集中与弹性模量差异相关,这一差异有助于驱动剪切带的形成和应力/应变诱导的马氏体转变。然而,如何协调界面在促进马氏体转变和剪切带形核两个看似矛盾的作用,仍是一个待解的难题。
Fig. 2 The relative distances among the compressive/dilatative transition, the exact interface and the interfacial maximum strain.
为了深入理解这一问题,由广东工业大学材料与能源学院付小玲副教授和加州大学伯克利分校Robert O. Ritchie教授领导的研究团队,采用分子动力学(MD)模拟,基于原子间势准确揭示了原子自发行为产生的非均匀弹性场,构建了不同尺寸的可转变球形B2纳米颗粒与非晶基体间的模型,从而更为精确地研究了界面弹性应力/应变场分布情况,为揭示该材料复杂的力学行为提供了新的视角。这个模型相较于传统Eshelby理论,提出了一个新的认识:在晶态-非晶界面附近存在一个纳米尺度的界面区,其中应变呈梯度变化,从压缩逐渐过渡到拉伸。在这一特定区域,研究详细描述了晶态-非晶界面、压缩/膨胀转换过程以及界面处的最大应变,而不是简单地将其归为常规的“界面”概念。
Fig. 3 The variations of total potential energy and volumetric stress associated with martensitic transformation behavior.
随着球形B2相沉淀物从小到大逐步增长,压缩/膨胀转换区域的位置也相应从非晶区内转移至B2沉淀物内部。界面处的应变状态也随之从压缩(对较小沉淀物)转变为膨胀(对较大沉淀物)。沉淀物和基体中的压缩(或膨胀)应力状态对马氏体转变的启动起到抑制(或促进)作用,并相应地增加(或减少)了转变的成核障碍。此外,B2相沉淀物与非晶基体间较松散(或紧密)的界面相互作用导致转变成核障碍的降低(或升高),进而影响材料的马氏体开始转变温度。最大的界面应变,与“界面应变/应力集中”密切相关,其实位于界面几埃以内的非晶基体中,而非精确的界面处。界面应力集中的数值明显高于精确界面的应变,并随着沉淀物尺寸的增加而增长。通过精准调控马氏体转变比例和界面应力集中度,可以大幅提升“转变介导的加工硬化和塑性”效应,有效解决即便在低温条件下也存在的强度与延展性的平衡问题。
Fig. 4 The innate interfacial strain gradient model for various sized precipitate enhanced metallic-glass composites.
该文近期发表于npj Computational Materials 9:226(2023),英文标题与摘要如下,点击左下角“阅读原文”可以自由获取论文PDF。
The innate interfacial elastic strain field of a transformable B2 precipitate embedded in an amorphous matrix
Xiaoling Fu, Yujun Lin, Mixun Zhu, Kai Wang, Jiaqing Wu, Xing Tong, Wenli Song, Ming Jen Tan, Yuanzheng Yang, Jun Shen, Gang Wang, Chan Hung Shek & Robert O. Ritchie
When a transformable B2 precipitate is embedded in an amorphous matrix, it is often experimentally observed that the crystalline-amorphous interface not only serves as an initiation site for the martensitic transformation due to local stress concentrations, but also as an inhibitor to stabilize the transformation, the latter being attributed to the “confinement effect” exerted by the amorphous matrix, according to the Eshelby solution. These two seemingly incongruous factors are examined in this study using molecular dynamics simulations from an atomic interaction perspective. An innate strain gradient in the vicinity of the crystalline-amorphous interface is identified. The actual interface, the compressive/dilatative transition, and the interfacial maximum strain are investigated to differentiate from the conventional “interface” located within a distance of a few nanometers. Our innate interfacial elastic strain field model is applicable for the design of materials with a higher degree of martensitic transformation and controllable stress concentration, even in cryogenic environments.
扩展阅读
媒体转载联系授权请看下方
最新评论
推荐文章
作者最新文章
你可能感兴趣的文章
Copyright Disclaimer: The copyright of contents (including texts, images, videos and audios) posted above belong to the User who shared or the third-party website which the User shared from. If you found your copyright have been infringed, please send a DMCA takedown notice to [email protected]. For more detail of the source, please click on the button "Read Original Post" below. For other communications, please send to [email protected].
版权声明:以上内容为用户推荐收藏至CareerEngine平台,其内容(含文字、图片、视频、音频等)及知识版权均属用户或用户转发自的第三方网站,如涉嫌侵权,请通知[email protected]进行信息删除。如需查看信息来源,请点击“查看原文”。如需洽谈其它事宜,请联系[email protected]。
版权声明:以上内容为用户推荐收藏至CareerEngine平台,其内容(含文字、图片、视频、音频等)及知识版权均属用户或用户转发自的第三方网站,如涉嫌侵权,请通知[email protected]进行信息删除。如需查看信息来源,请点击“查看原文”。如需洽谈其它事宜,请联系[email protected]。